Digital piezo nanopositioning controllers have a reputation for being expensive and only cost efficient in high-end applications. A recent new design approach has changed this situation, making advanced digital control features affordable for main stream piezo motion applications.
History of Digital Motion Controllers
Position control for magnetic motors has been almost exclusively digital for nearly three decades. In the mid-1980s, digital motion control chips began to be available, such as the pioneering HCTL-1000 chip from HP. These chips were easy for controls engineers to interface with the position feedback encoders of the era that provided simple, incremental quadrature signals suitable for digital processing. Also, for motors, the drive signal is a velocity or force command, so only low output resolution was necessary, regardless of axis travel. 10 bit analog or 8 bit PWM output resolutions were common, and these were very tractable for controller designers to employ in cost-effective designs. With the exception of analog tachometer feedback integrated as a supplementary loop for higher-performance systems of that era, magnetic motor control has been virtually all-digital ever since.
Why Piezo Nanopositioning Control is Different
In the world of piezo nanopositioning systems, the situation was and remains quite different. The purpose of these systems is to provide extremely high position resolution in the nanometer and sub-nanometer range with very fast response in the sub-millisecond range. Here, the position feedback is traditionally provided by analog, absolute measuring sensors (strain gauges, piezo resistive gauges, capacitive gauges or LVDTs). For piezo stack actuator-driven positioners, the drive signal from the controller operates in the position domain rather than representing a velocity or force command as is the case with magnetic motors. Consequently, any electrical noise or drift of the drive signal results in an unwanted position change.
Note: Piezo-stack driven nanopositioning systems are not to be confused with piezo motor positioners, which provide longer travel ranges and have more similarities with traditional motorized positioning systems. For more information on different types of piezo motors read the piezo motion tutorial.
This necessitates high-quality, low-noise, low-drift circuit design for the mission-critical servo circuit. In particular, the coarse DAC or PWM designs common in motor controllers were inappropriate for nanoscale piezo positioning. In addition, the high responsiveness of piezo mechanisms necessitates very fast processing that traditionally was too costly for all but the most elite nanopositioning applications.
This is why most affordable nanopositioning controllers have historically utilized analog servos. A microcontroller driving a digital-to-analog converter (DAC) was often added to provide a display and perhaps simple interfaces for computer commandability, but the critical servo-control and linearization functionality still relied on legacy, fixed-function analog circuits. Calibration and tuning for significant load changes required careful adjustment of tiny trim-pots using precision external metrology. Linearization could accommodate second-order sensor nonlinearities at best, but drift in the internal or external DAC would cause an unwanted positional change.
Effects of the interaction of the PZT ceramics, preload, flexures, and couplings show up in the graph and the result is different from a straight line.
Still, these controllers remained the affordable, robust workhorses of nanopositioning for both research and industrial applications.
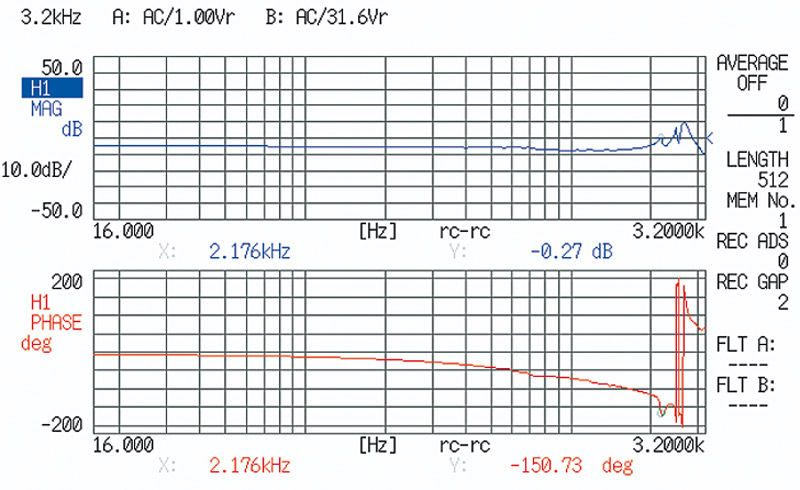
PI’s implementation was unusual in that even its most affordable analog nanopositioning controllers implemented a notch filter. This desensitizes the servo to resonances in the nanopositioning mechanism, allowing the servo to run at high gain for unusually good positioning throughput.
All-Digital Started with High End Applications
All-digital piezo controllers have existed since the early 1990s, but they tended to be costly. These units replaced the analog servo-control circuit with a fast, real-time digital loop that integrated the DAC, providing automatic stabilization to a previously unachievable degree. These controllers quickly became the default for drift-intolerant applications in single-molecule biophysics, atomic force microscopy and semiconductor microlithography. These controllers quickly surpassed the 16 bits resolution common among prior-generation instrumentation-quality DACs. Suddenly, 18 to 21 bit positional resolution could be achieved, sometimes even more. This, in turn, facilitated the development of really long-travel piezo flexure guided stages that retained fast nanoscale positionability, even with travels exceeding 1mm.
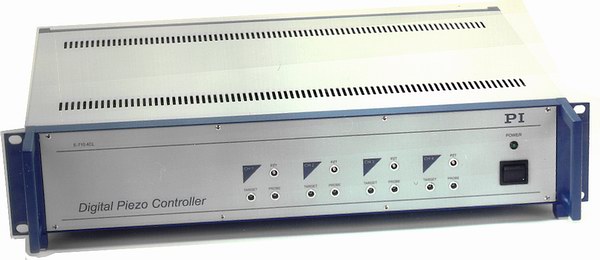
The functionality of digital nanopositioning servo controllers quickly thrived in novel, application-enabling ways. Data recorders and waveform generators were integrated; advanced high-order linearization broke new ground for nanoscale accuracy. Advanced new servo techniques were just a firmware update away, facilitating novel tracking applications and implementing advanced settling-time-reduction algorithms. More information on advanced piezo control algorithms is here. The addition of a second notch filter helped passify challenging loads. And the integration of calibration “ID chips” in the nanopositioning mechanisms eliminated the need to keep controllers and mechanics as a matched set.
Design Advances Make Digital Nanopositioning Control Affordable for Main Stream Applications
Four decades of piezo controller design leads to advances and new ideas of approaching a problem. The recent introduction of PI’s E-709 (single axis) and new E-727 (multi-axis) digital controllers bring the benefits of true-digital design to highly affordable price points. Like all true-digital controllers;
- The digital-to-analog converter (DAC) chip is internal to the servo loop rather than external, facilitating stability, high-order linearization and better resolution.
- The calibration and tuning is accomplished via software, with no trim-pots.
- Communications interfaces of extraordinary speed are standard.
- Broad and deep software libraries are provided, allowing rapid, multi-platform application deployment and ready access to advanced functionality.
- Waveform generators and data recorders are integrated.
- ID Chip functionality is supported, allowing positioners to be mixed and matched with automatic calibration.
- High-performance, high-efficiency amplifiers are built in, providing a compact, single-box solution.
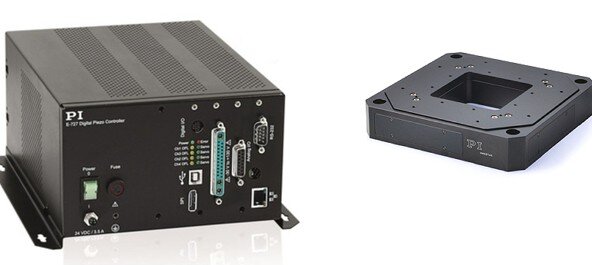
In Detail: Analog vs. Digital Piezo Control
Traditional (analog) nanopositioning controller designs utilize sensitive op-amp circuits to implement the familiar and robust Proportional-Integral feedback-driven servo. The job of the servo is to reduce the difference between the command signal (generally a voltage generated by a DAC elsewhere in the controller or on a card in the user’s PC) and the feedback signal from the position sensor embedded in the motion device.
The most sophisticated examples of this popular architecture integrate features, such as:
- Sensor linearization, which improves the absolute accuracy of the motion system.
- Piezo relaxation compensation, which helps ensure crisp pull-in and stable position-hold in point-to-point operation.
- A notch filter, especially valuable in high-dynamic applications since they desensitize the servo to the mechanism’s observable resonances and thereby allow higher-gain operation. These are standard features of all PI analog servo electronics.
However, in this time-tested architecture, any change in the DAC’s output will drive a position change. This is of course very desirable behavior, but if the DAC drifts or noise enters the system through its connection, the result will be position instability.
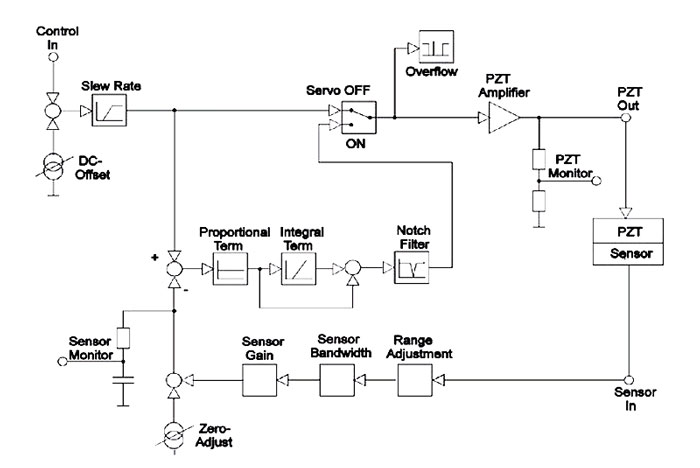
By comparison, digital controllers implement their servo functionality in a DSP rather than in analog circuitry.
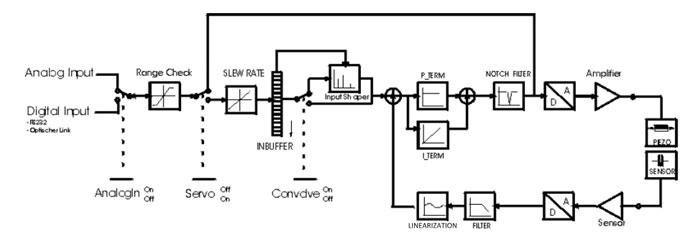
This true-digital architecture allows the DAC to be integrated inside the servo loop. The best implementations provide sensor linearization up to 4th or 5th order, versus 2nd order for the best available analog designs. And DAC drift can be virtually eliminated since the system intelligence can differentiate between desired and undesired motions, as detected by the feedback sensor. This in turn enables integration of high-bitness (but sometimes drifty) DACs for finest resolution.
Note: While many analog piezo controllers add a microprocessor and DAC on top of a conventional analog servo circuit in order to satisfy user demands for computer interfaceability using USB, RS-232 or similar industry-standard interfaces, some suppliers misleadingly call these “digital” controllers, but their servo functionality remains analog.
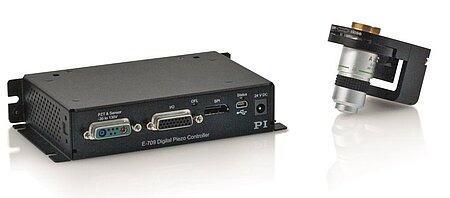
Driving a Savings Cascade for High Volume Applications
For the first time, breakthroughs in digital design have allowed commercialization of a digital piezo nanopositioning controller at the same price as conventional analog controllers. PI’s E-709 also was the first digital piezo controller to accommodate cost-effective strain gauge sensors for positional feedback. These sensors are based on the strain of metal foils or semiconductor films (piezoresistive sensors) and are used when space or cost limitations prevent the use of more advanced capacitive sensors, or where the requirements in terms of resolution or thermal sensitivity are not as critical. (E-709 and E-727 are, of course, also available for use with capacitive sensors for applications requiring the utmost in resolution, accuracy, EMI resistance, bandwidth, and stability.)
This means the nonlinearity of strain sensors can now be affordably addressed by real-time digital compensation, providing up to a 10X improvement in absolute accuracy.
Parameter Changes On the Fly: Quicker Set-Up, Increased Flexibility
Changing the gain settings of an analog nanopositioning servo (and the center-frequency of its notch filter, if so equipped) required physical access to trim-pots internal to the controller. These settings should be optimized for the system’s load. If the configuration changes significantly, re-adjustment is good practice, but access hassles, time costs, and the availability of skilled technicians sometimes prevent this. For example, a sophisticated optical apparatus with a piezo-based focusing mechanism like a PI PIFOC might be used with several microscopy objectives of significantly differing mass. In such situations, a compromise setting was traditionally used to ensure stable operation. This can impose a trade-off in responsiveness.
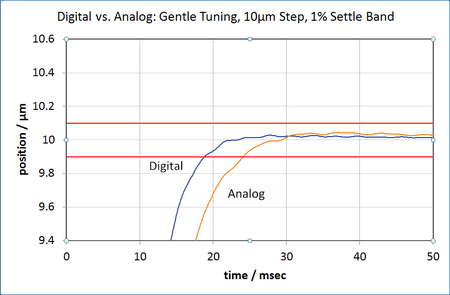
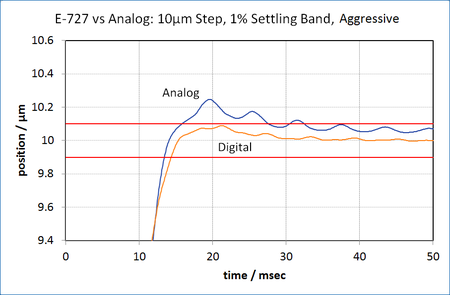
By comparison, true digital controllers — including E-709 and E-727 — offer dynamic settings that can be easily changed remotely via software and then safely stored to the unit’s flash RAM. In the microscopy example just mentioned, optimized dynamic parameters can be downloaded to the controller at any time without requiring physical access to the unit or even a power-cycle.
This capability allows easy optimization for momentary application requirements in addition to configuration changes, such as a new load. In OEM applications, the tool’s embedded PC can download preconfigured settings on-the-fly and without pause to customize the system’s dynamical behavior to the requirements at that moment. The figure below for example, shows two settings for the same load: a fast motion of less than 5msec, and a gentler motion of the same amplitude. The faster, crisper motion might be desirable for time-critical applications like optical sectioning and nano-patterning, whereas the gentler motion might be more suitable for biological samples or surface-following probing applications like AFMs and nanotribology scanners.
These units are also unique among affordable nanopositioning controllers in providing two notch filters, allowing high-dynamic operation in situations where motion-driven ringing in the motion device and supporting structure would otherwise be problematic and require lower, less responsive gain settings.
E-709 provides advantages for autofocus applications, such as used in microscopy, industrial inspection, scanning, and genomics applications. It can interface in real time laser focus sensors, providing stable positions on the order of tens of milliseconds. It provides responsive real-time tracking, and it supports PI’s Fast Focus & Freeze capability, where the unit can be bumplessly switched from an external (focus) sensor to a position sensor in the piezo mechanism, allowing precise, high-stability motion with respect to the focal plane.
Interfacing Speed
E-709 and E-727 provide a wealth of interfacing capabilities together with highly optimized software building-blocks. To begin, there are responsive USB, RS-232, and SPI interfaces on both. High-speed TCP/IP Ethernet connectivity for easy integration into automation and remote-access architectures from lab to fab is available on the E-727. Waveform generation and data recording capability are built-in and facilitate repetitive scanning and similar applications, offloading position generation from the host.
These controllers offer three real-time interfaces:
- Analog I/O interfaces, sampled/updated at the full servo cycle rate, provide easy integration with National Instruments’ multifunction cards, among other external command-signal possibilities. LabVIEW® users can even leverage this interface using PI’s Analog GCS (General Command Set) library — the industry’s first plug-and-play library for analog nanopositioning.
- A standard high-speed SPI interface offers the ability to communicate digitally using industry-standard physical protocols. You can command and interact with the systems servo update rate with no risk of loss or noise pickup in cabling.
- TTL I/O functionality allows triggering and synchronization with other host processes. These controllers utilize the same PI GCS command set as all PI controllers. This means programmers can write consistent code for coarse/fine applications, with the coarse positioner (like the ceramic motor-driven, self-clamping U-751/M-686 microscopy stage and its C-867 controller). Included with Nanopositioning controllers is a wealth of software tools ranging from an extensive LabVIEW® library, to an industrial-class Windows .dll and Linux .so libraries. A graphical setup and exploration software, called PIMikroMove, helps with system startup.
Special OEM Configurations
These digital nanopositioning controllers are also available without a case for embedded OEM applications. A version with only an analog position-command interface (E-609) offers special economy for backwards-compatibility applications, formerly served by analog controls.
Reference Class Applications
Controllers such as the E-727 and E-709 can handle most tasks precision motion engineers encounter in industrial and scientific nanopositioning applications. For situations with the most demanding requirements, a controller with a different architecture is also available. With more processing power and an even faster servo loop (50kHz), the E-712 modular controller can perform complex tasks such as automated multi-channel, multi-axis photonics alignment, coordinated motion of multiple piezo elements such as found in PiezoWalk® nanopositioning actuators and ultra-fast servo applications such as required for track following servos in high-speed read/write head testing.
Conclusion
Digital nanopositioning controllers help advance the field of nanotechnology. By providing features and capabilities formerly reserved for controllers costing many times their price, the E-727 and E-709 family enables a host of new research and industrial/OEM applications. OEMs will value their quick setup and remote customization capabilities combined with their power, space-saving design, and high responsiveness. Even their cabling scheme is targeted to make the OEM’s job easier and more productive. Meanwhile, research users will prize their stability, performance, wealth of supporting software, interfacing flexibility, and ease of use.
Blog Categories
- Aero-Space
- Air Bearing Stages, Components, Systems
- Astronomy
- Automation, Nano-Automation
- Beamline Instrumentation
- Bio-Medical
- Hexapods
- Imaging & Microscopy
- Laser Machining, Processing
- Linear Actuators
- Linear Motor, Positioning System
- Metrology
- Microscopy
- Motorized Precision Positioners
- Multi-Axis Motion
- Nanopositioning
- Photonics
- Piezo Actuators, Motors
- Piezo Mechanics
- Piezo Transducers / Sensors
- Precision Machining
- Semicon
- Software Tools
- UHV Positioning Stage
- Voice Coil Linear Actuator
- X-Ray Spectroscopy