Why Fast Steering Mirrors are used in Laser Communication / Free Space Optical Communication
High Precision Tip/Tilt Mechanisms Keep Laser Beams Stabilized and Precisely on Target
Driving the first photonics boom of the late 1990s was the initiative to replace costly, laggy, and limited satellite telecom links, based on radio frequency (RF) signals with long-haul optical fiber cables running across oceans and continents. Readers of a certain age will remember the days when a long-distance phone call was not only costly but annoying: there was often a perceptible pause between a caller’s utterance and its delivery to the recipient, and vice versa. This was because the call was routed via satellite and the geostationary satellites like 1962’s Telstar1 were so distant that the transit time from earth base-station to satellite and back was easily perceptible. Capacity was limited, per-minute pricing was high, and data-rates as we have come to think of them were truly in the Stone Age - lasers and fast steering mirrors played no part in telecommunication back then.
Optical Fiber Networks
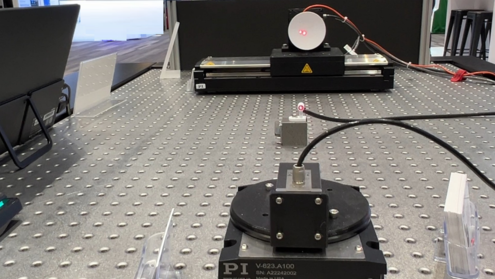
Optical cabling girdling the planet quickly replaced these fragile and problematic links with the capacious and speedy global network we enjoy today. This was truly a key enabler for the deployment of the Internet: the photonics-based physical spiderweb that—among so many other things—underlies and implements the World Wide Web that brings this article to you.
Today, we are seeing the first glimmers of a phenomenon that, in a sense, brings Earth’s global communications infrastructure full circle: the implementation of a vast space-based network of new-generation communication nodes. These nodes are compact satellites, deployed by the thousands to girdle the entire planet. They link not only to ground stations but to each other, promising to route data efficiently and rapidly from any point on the planet to any other. The vision is to provide every car, every piece of physical infrastructure, every shipping container and tractor-trailer rig, even every cow with connectivity to enable a spectrum of fantastical applications.
Free Space Optical vs Radio Frequency (RF) Communication
Due to the limited bandwidth, as well as RF signals’ lack of precise focusability, laser beam-based optical communications have been developed to complement traditional satellite communications. Not only do they provide higher throughput, but they also require less power (satellites have no access to the grid). In addition, they offer higher security, since the optical signal is highly focused, cannot be eaves-dropped on easily, and the straightforward propagation reduces the risk of interference, a phenomenon very common in the radio frequency range.
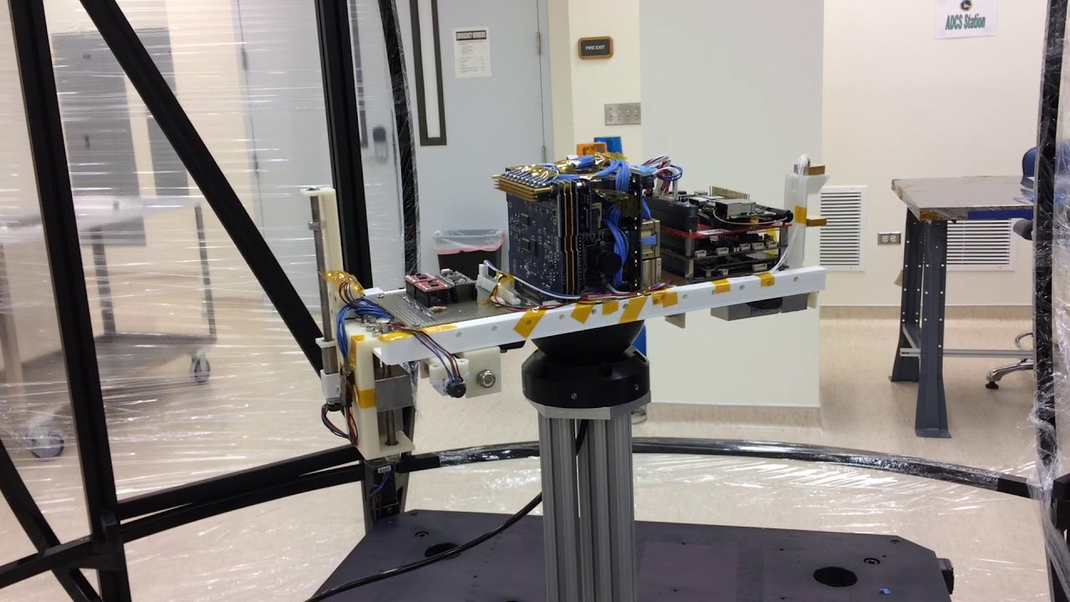
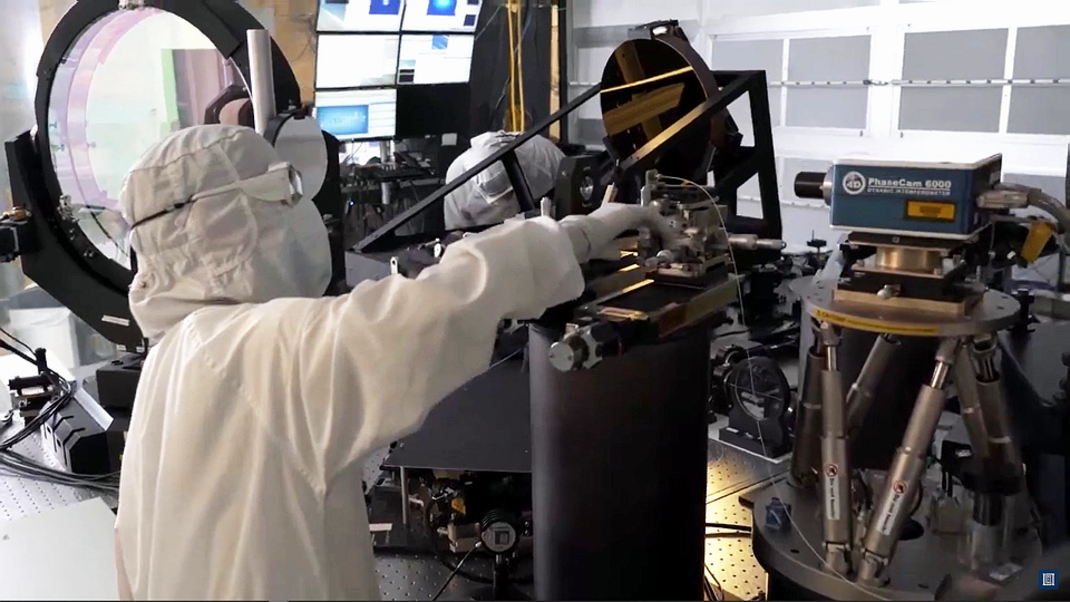
The inter-satellite links are via gossamer laser beams, with each satellite tracking and linking to its neighbors. Among the leaders in the field is SpaceX, and the first test launches of its laser-equipped satellites occurred recently, with founder Elon Musk verifying on social media that these launches of 142 satellites in a single payload included their first implementation of the company’s inter-satellite laser links 1 2. The complicated link topology has been reduced to a YouTube video3 and interested readers will find many resources for exploring the approach available on social media4. Other players include Amazon, Momentus, Fraunhofer IOF, Arribada Initiative, Outernet, and Lacuna Space. Sub-orbital approaches to blanketing the Earth with bandwidth have been mounted by Google’s balloon-borne Project Loon5, Facebook’s canceled drone-based Project Aquila. These also leverage laser-based interconnect approaches.
Importantly, these direct interconnects avoid the crowded-spectrum issue that impedes advancing applications of broadcast approaches, along with vexing regulatory strictures and delays.
And while optical communication already provides much higher bandwidth compared to RF, a new approach, based on orbital angular momentum could further expand the capacity of free-space optical communication.
Deep Space Optical Communications (DSOC)
According to NASA, “Future human and robotic expeditions into deep space must count on the fastest, most efficient means of communicating with mission managers on Earth.”
Here, the goal is to provide a stream of HD video, in addition to the necessary operating parameters, required for mission-critical decisions across the vast distances of the solar system. Laser communications with data rates up to 100X the current rate is targeted without increasing size or power consumption. However, while laser light can carry significantly more information than radio frequency transmissions - more than even the next generation 6G Terahertz mobile communication standard based on millimeter wavelengths can provide - its propagation speed is bound by the same physical laws, meaning real-time communication to the end of the solar system is a relative term, and on-board artificial intelligence is required to make quick decisions. More on NASA and DSOC.
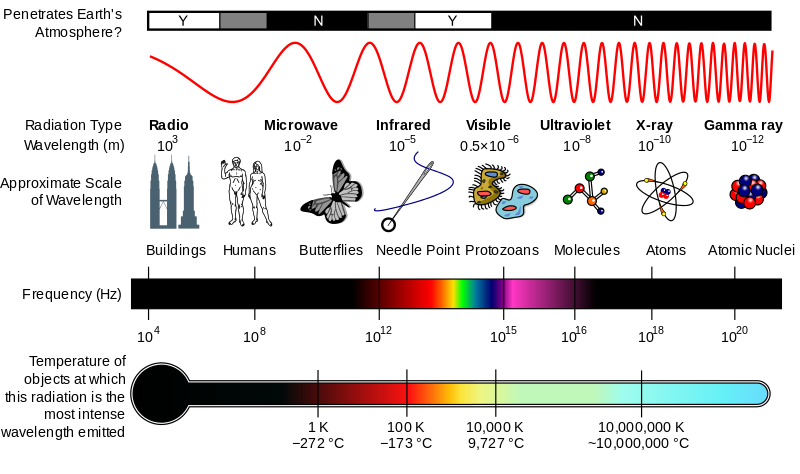
Fiberless Photonics on Earth
Back home on earth, a related approach to provisioning point-to-point networking using “fiberless photonics” is being rebooted. The first commercial attempts at terrestrial free-space optical communications were attempted in the late-1990s photonics boom, driven by innovators like Terabeam, Optical Crossing, AirFiber, and Lucent’s OpticAir. Unfortunately, these enterprises suffered the same fate as so many others when the photonics boom—driven as it was at the time by the single application of long-haul telecom—entered a deep slumber after capacity needs had been met for the foreseeable future by the laying of “dark fiber” for eventual provisioning. Those days are past. The industry awakened to exponentiating demand for data driven by a host of applications from social networks to streaming media, personal medicine to the Internet of Things, Industry 4.0 to autonomous vehicles.
Despite the temporary harrowing of the industry in the decade following 2002, the principle behind free-space optical communications remained sound, and it is fundamentally similar to optical telegraphy dating to the 18th century7 (though of course much faster): messages are encoded in blinks of light conveyed from one terrestrial location to another. This presents the potential for rapidly provisioning secure, dedicated photonic interconnects between locations, such as from building to building in a crowded city, or for the “last mile” of a broader network.
Twenty years ago, these interconnects required substantially expanded beams—telescopes, typically on the order of 200mm aperture—both for fault-tolerance against the occasional bird breaking the beam and for eye-safety. Today, a new wave of innovators is leveraging this era’s more advanced error-correction algorithms and more-efficient electronics to allow lower-cost yet higher-speed implementations. Transcelestial of Singapore is an example, offering compact, pole-mounted transceivers with up to 10GBps throughput.
Why Fast Steering Mirrors?
Due to the low divergence of laser beams, exact pointing solutions must be found to keep the beam exactly on target over the vast distances covered in satellite-to-satellite links, ground-to-satellite or deep space communication. In addition to a coarse steering system, which can be achieved by the satellite attitude system, a high speed fine steering system is required to deal with vibrations from the satellite (e.g., stabilization systems).
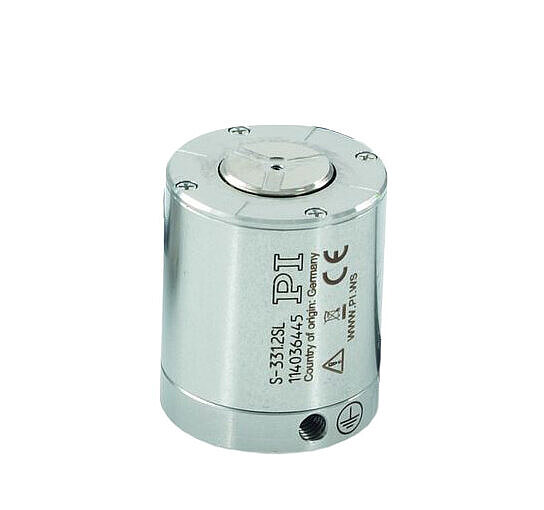
In earth to satellite communication, atmospheric turbulence is another factor that can shift the beam from its original path. At the receiving end, the laser beam is coupled into a single mode fiber which requires very high accuracy to avoid optical power loss. Piezoelectric or electromagnetic fast steering mirrors (FSM) can provide angular resolution down to the nanoradian range, with mechanical bandwidth up to the kHz range. They are compact, fast, and accurate enough to deal with the usual disturbances in these applications. While piezo-driven FSM’s provide higher resolution and bandwidth, electromagnetic units (usually voice coil driven FSM’s) allow for larger steering angles.
Since the 1990s, PI’s fast steering mirror technology has been utilized in both terrestrial and space-based testing and implementation. Offering efficient and fast designs based on piezoelectric or electromagnetic actuation, PI’s solutions include a variety of publicly-available off-the-shelf laser beam stabilization products and confidential custom offerings, with more to come.
If you need to tap into the knowledge of our engineering teams on fast steering mirrors, please do not hesitate to contact us.
More information on »optical communication and space lasers
1 Wikipedia entry on Telstar: https: //en.wikipedia.org/wiki/Telstar
2 Elon Musk on Twitter: https: //twitter.com/elonmusk/status/1353408098342326276?s=20
3 https: //wccftech.com/spacex-starlink-satellite-laser-test/
4 SpaceX StarLink routing topology visualized: https: //www.youtube.com/watch?v=AdKNCBrkZQ4&feature=youtu.be
5 Online and social media resources include Wikipedia (https: //en.wikipedia.org/wiki/Laser_communication_in_space) and Reddit (https: //www.reddit.com/r/spacex/)
6 https: //loon.com
7 Example discussion of optical telegraphy: https: //www.google.com/books/edition/Communications/7eUUy8-VvwoC?hl=en&gbpv=1&pg=PA29&printsec=frontcover
Blog Categories
- Aero-Space
- Air Bearing Stages, Components, Systems
- Astronomy
- Automation, Nano-Automation
- Beamline Instrumentation
- Bio-Medical
- Hexapods
- Imaging & Microscopy
- Laser Machining, Processing
- Linear Actuators
- Linear Motor, Positioning System
- Metrology
- Microscopy
- Motorized Precision Positioners
- Multi-Axis Motion
- Nanopositioning
- Photonics
- Piezo Actuators, Motors
- Piezo Mechanics
- Piezo Transducers / Sensors
- Precision Machining
- Semicon
- Software Tools
- UHV Positioning Stage
- Voice Coil Linear Actuator
- X-Ray Spectroscopy